
Unmanned Aerial Vehicle
Meet Millie 2025
Millie is McGill Robotics’ newest drone, aiming to combine fixed-wing flight with quadcopter hover capabilities through a custom hybrid frame.
Identifying drop zones, delivering payloads safely, and avoiding other drones and obstacles, McGill Robotics’ Millie will be the most capable McGill Robotics drone to date.
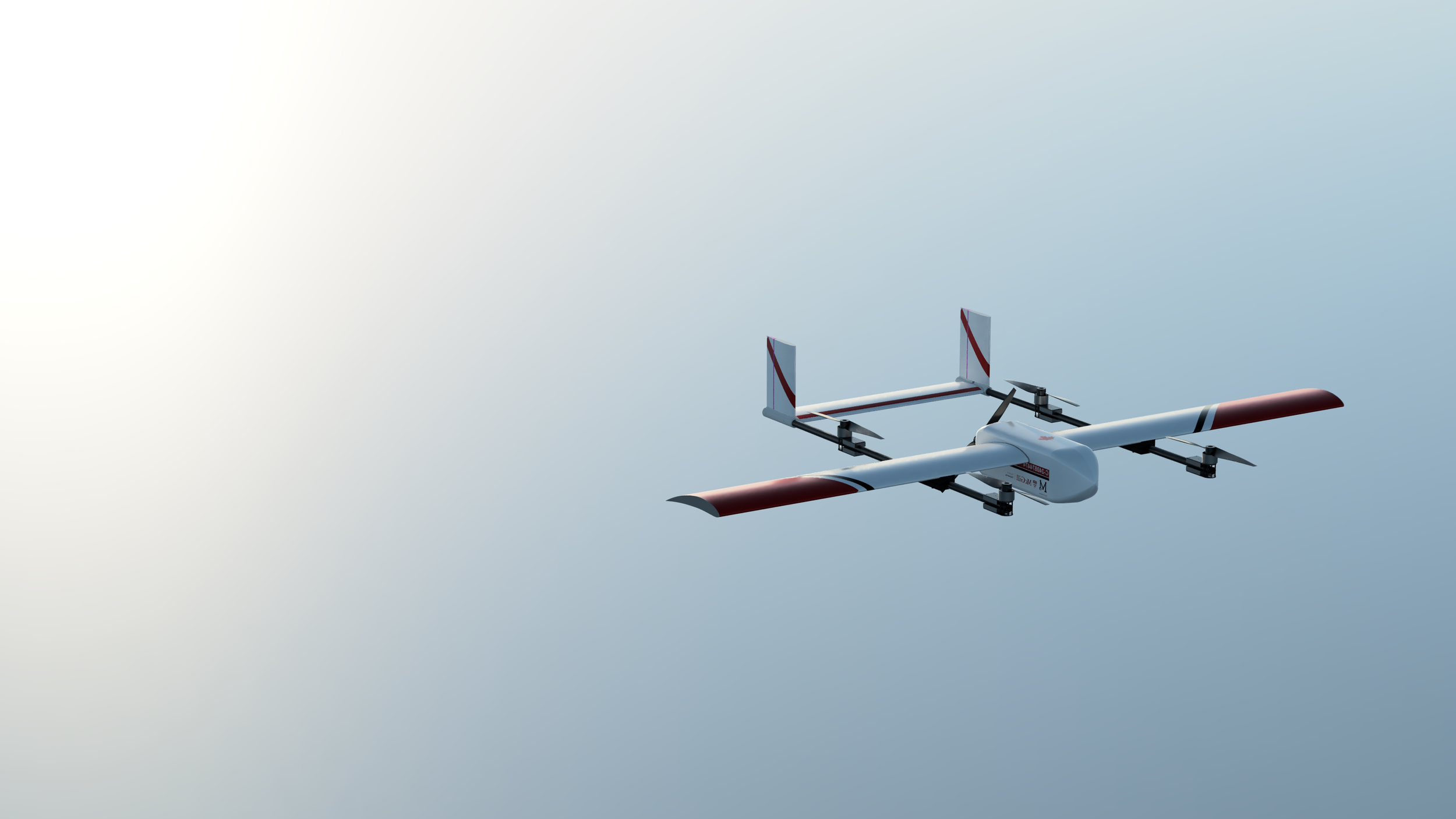
Meet Our Team
Over the course of the school year, our team of talented engineers has worked tirelessly to produce a drone to represent our team at this year’s Student Unmanned Aerial Systems Competition. Spanning three different sub-divisions of Mechanical, Electrical, and Software, the students who bonded together to develop and design Millie are truly some of the most dedicated and exceptional students that McGill has to offer.
38
Members
4th in mission demonstration at SUAS 2024
7
Leads and PMs
Millie at a Glance
Autopilot
CUAV x6+
Power System
12V 4500mAH Tattu LiPo Batteries (x6)
Propulsion
T-Motor V505(x4)
T-Motor AT5220A 20-25CC VTOL motor
T-Motor ESC FLAME 60A12S V2.0 (x5)
Compute Module
NVIDIA Jetson Orin Nano
Software Stack
Robotics Operating System (ROS 2) Humble
Ubuntu 22.04 LTS
Python3, micro-ROS
Computer Vision
Raspberry Pi High Quality Camera
Mechanical Design.
In the mechanical division, we strive for continuous improvement of the structure of the drone. The designs undergo multiple iterations of CADing, prototyping, and testing before being implemented into the final product. Simulations also constitute an integral part of the design process to verify that the components meet our requirements and ensure good integration with the work of other divisions. We use a variety of technologies, such as plastic and metal 3D printing and carbon fiber casting, to optimize the functionality of the drone. Through the collective efforts of the whole division, we aim for maximum mobility, durability, and stability throughout the competition.
Wings
For this year’s competition, we have adopted a new airfoil shape, the NACA 8412 airfoil, which has been selected based on its high lift coefficient, low drag characteristics, steady moment coefficient over a wide range of angles of attack, and gentle stall profile.
The process for manufacturing the wings involves creating the foam core of the wings using hot wire cutting or machining, followed by resin and fiberglass layup with embedded Kevlar strips at control surface locations and then vacuum bagging for 24 hours to allow ample time for curing.
Fuselage
The fuselage is composed of longerons, frames, spars and floor and wall boards, similar to the construction of commercial aircraft fuselages. Millie’s fuselage is made of laser-cut plywood and carbon fiber sheets. Super glue and DP420 was used to ensure the pieces stuck together firmly. For added stiffness and to seal the fuselage, a 2 part fiberglass shell was glued on the frame.
Inside the fuselage, the batteries sit at the front in their own section, with 2 frames surrounding them. Behind the batteries are 5 frames, creating 4 sections. These 4 sections house the electronics, namely, the ESCs, the Jetson Nano, and the autopilot. The payload system is attached to the underside of the fuselage
Electrical Design.
The Electrical Division focuses on battery design, communication hardware, and power management and distribution. The system includes student-designed PCBs and firmware to allow the different subsystems to function seamlessly with a Jetson computer. Members on the electrical division develop circuit design skills using Altium, through hole and SMT soldering skills, and embedded software development skills.
Software Design.
The software division is responsible for designing the computer vision framework, a precise control system, and communication between the team on the ground and electrical components, as well as an obstacle detection and avoidance system while in the air. Next, they develop comprehensive simulations that test various systems such as mission planning, vision, controls etc., to ensure the reliability of the system.
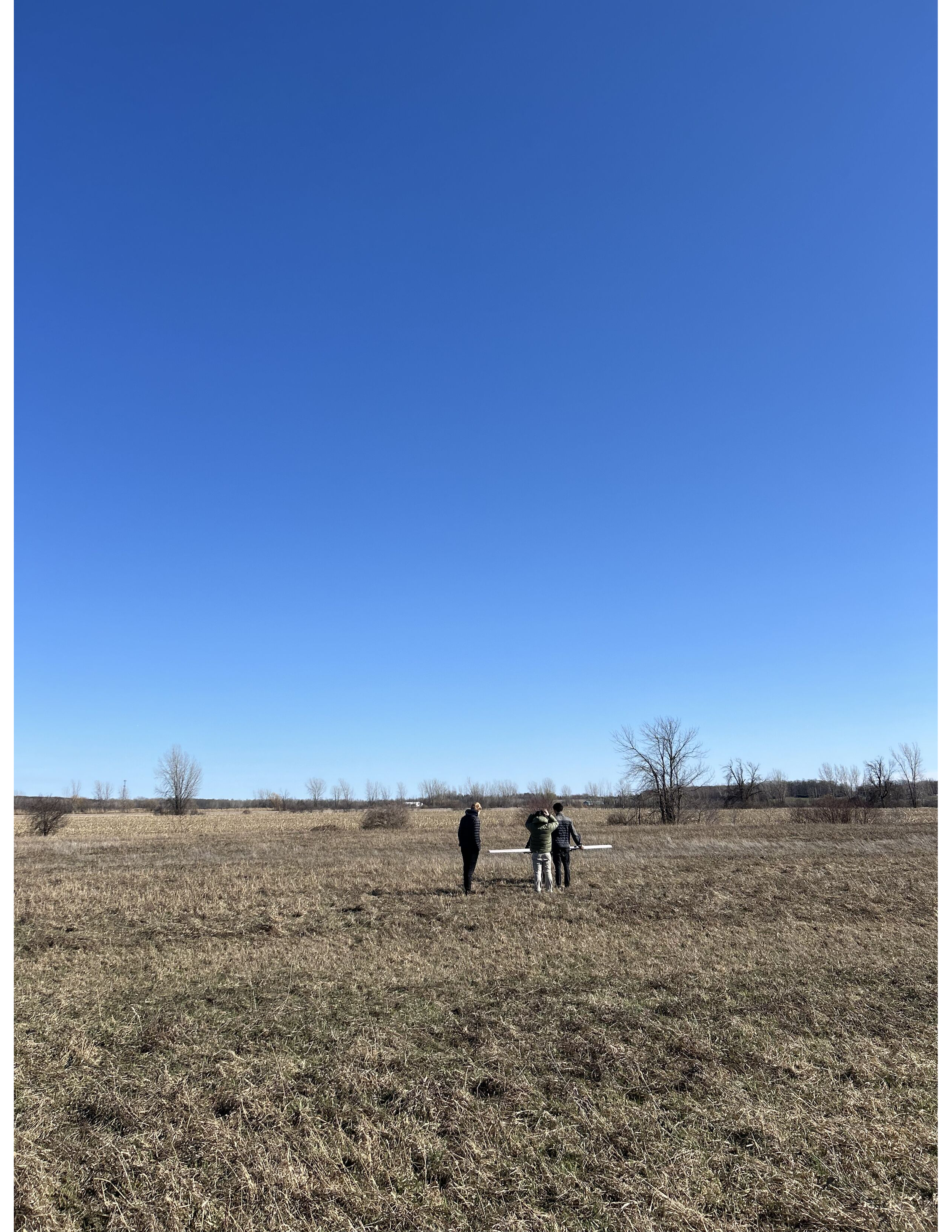

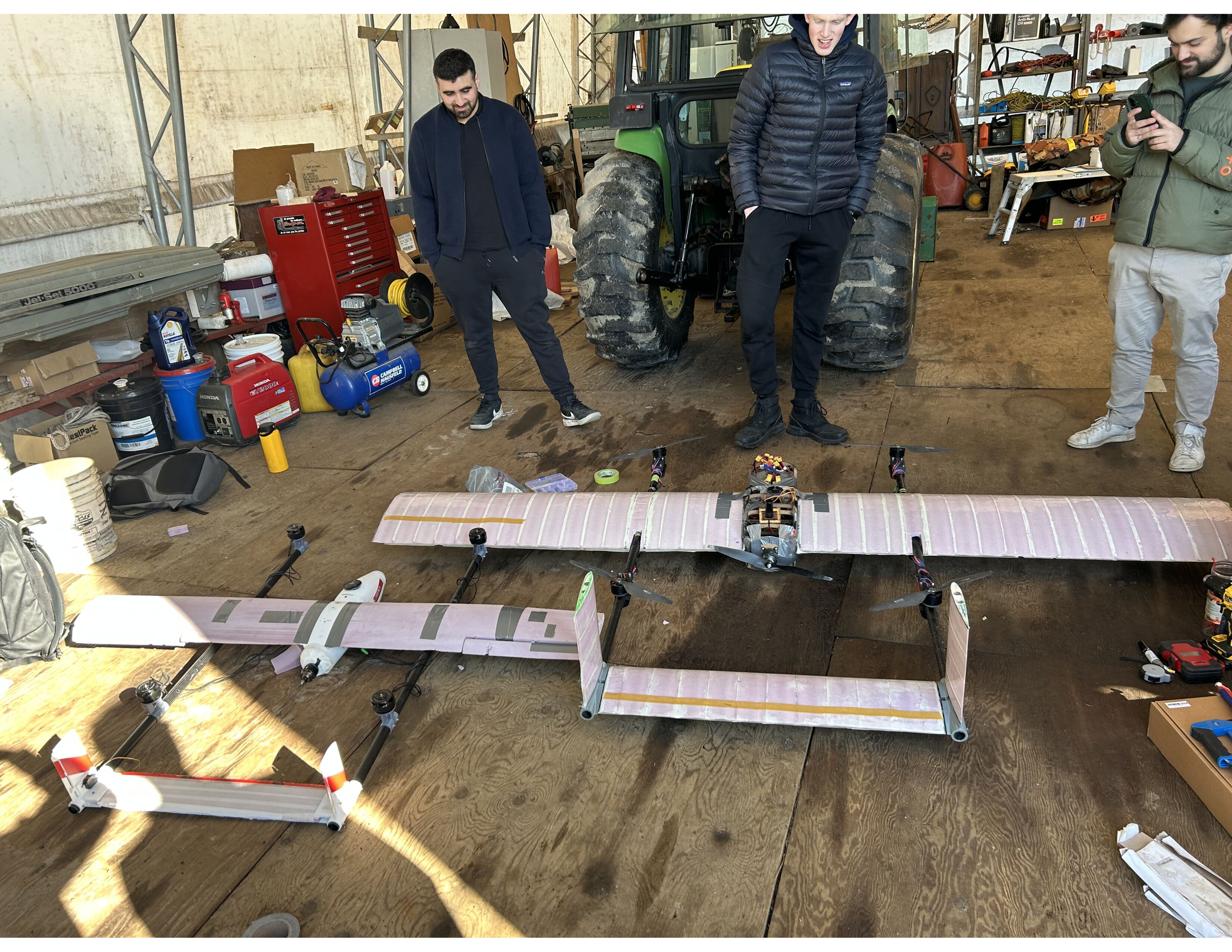

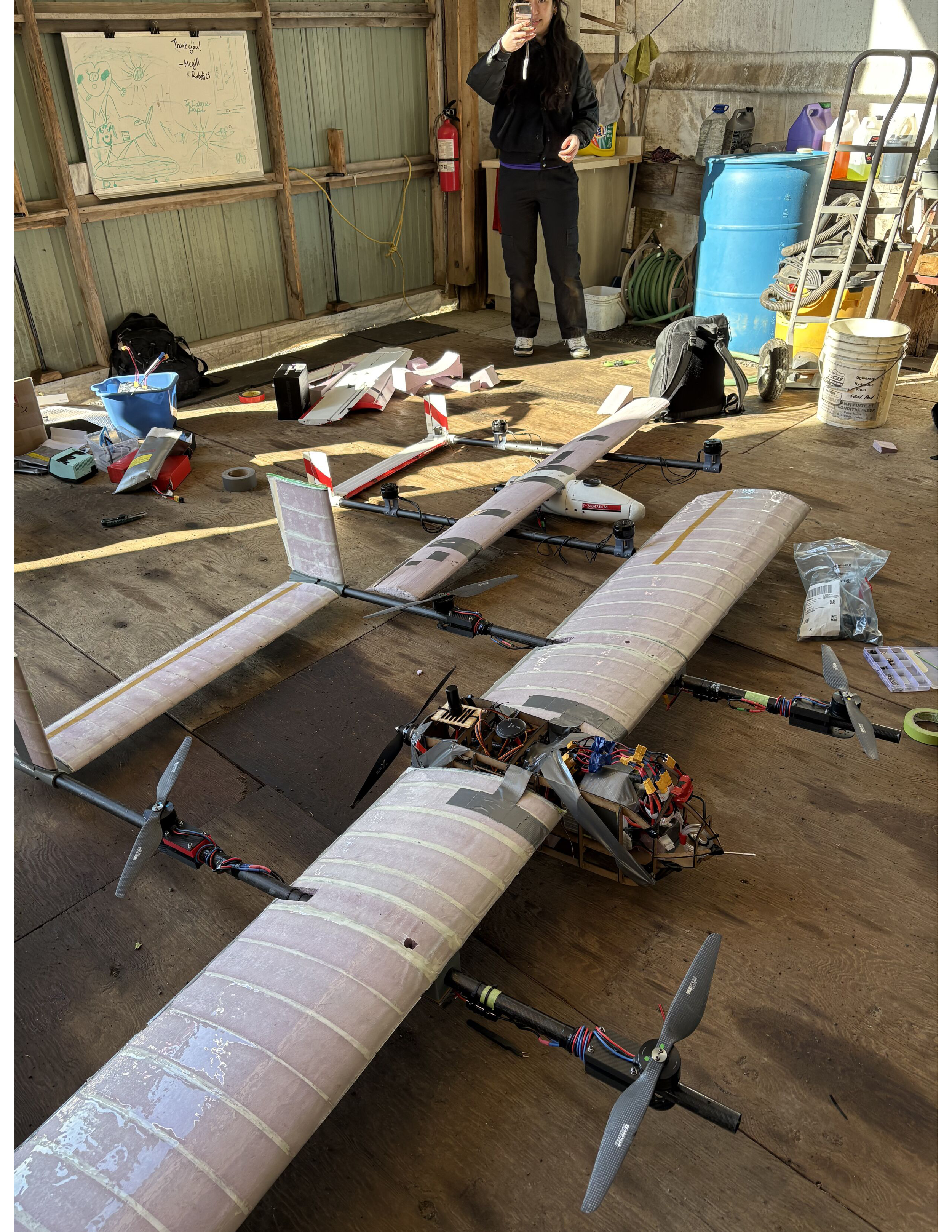
Our 2025 SUAS Proof of Flight Readiness
Meet the Drone Team
Community & Outreach at McGill Robotics
McGill Robotics mission is to inspire students to build robots and to build robots that inspire students. We believe it is important to give back to the community which is why we are an organization that advances the field of robotics but also nurtures a community that values sustainability, collaboration, and education.