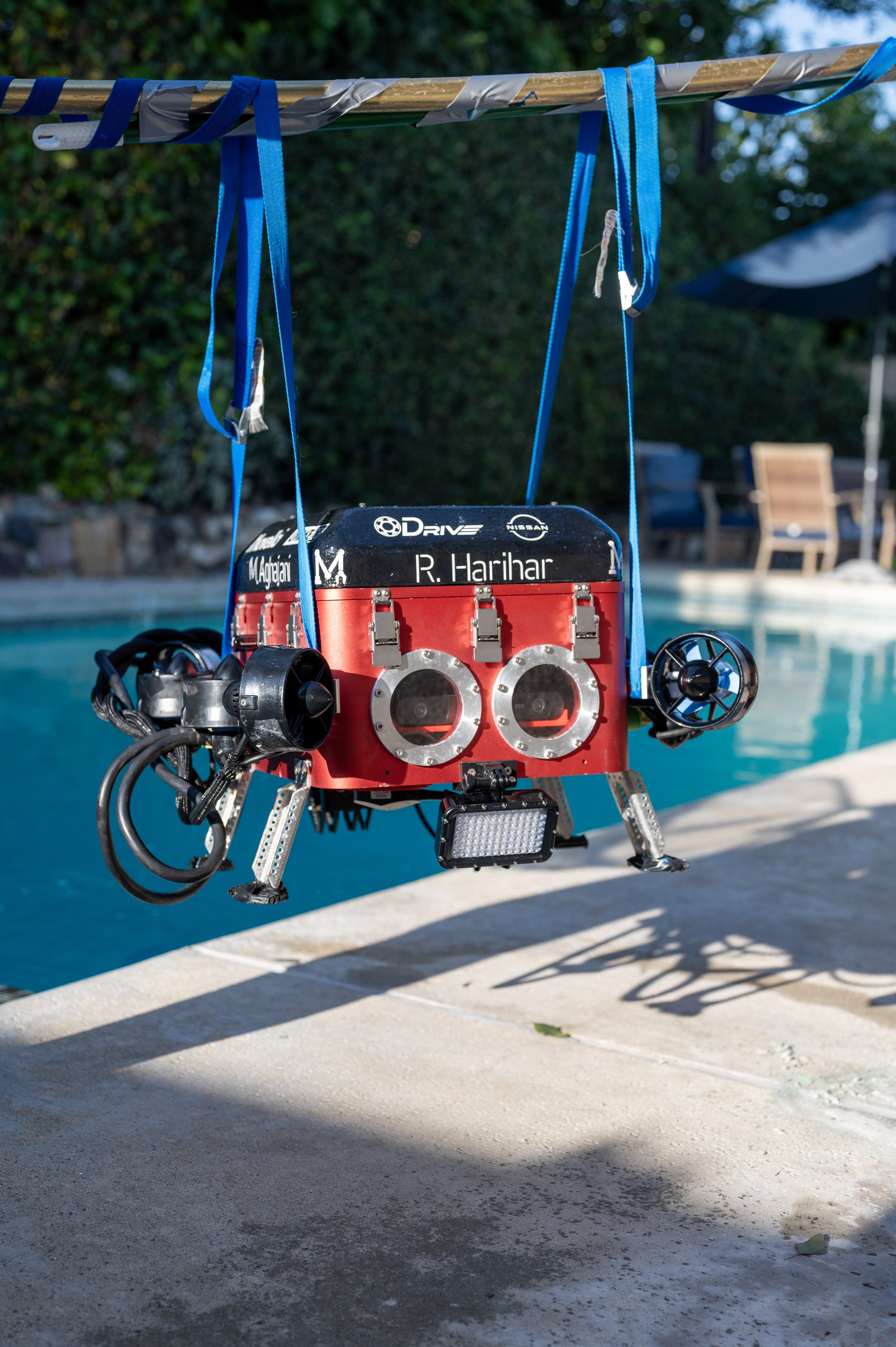
Autonomous Underwater Vehicle
Meet Douglas
The McGill Robotics Autonomous Underwater Vehicle (AUV) Team presents Douglas, a completely redesigned AUV developed during the 2023-2024 scholastic year. Douglas features an entirely new mechanical body consisting of a hull, chassis, and grabber and dropper mechanism. Our electrical team has re-imagined the internal electrical architecture and has implemented a new revision of the power distribution board, custom-designed display, and actuator boards. The software team improved our core software functionalities including a more precise navigation algorithm, development of advanced vision, and simulation algorithms
Meet Our Team
Throughout school year, our dedicated team of engineers has worked tirelessly to bring Douglas to life, our Autonomous Underwater Vehicle (AUV) representing us at this year’s RoboSub competition. Spanning three different sub-divisions of Mechanical, Electrical, and Software, over 40 students bonded together to develop and design Douglas. Their commitment and excellence truly showcase the best of McGill’s student talent.
64
Members
4th in presentation at RoboSub 2024
8
Leads and PMs
Building Robots For A Sustainable Future
In partnership with McGill’s Sustainability Projects Fund, McGill Robotics is developing robots to assist researchers in large-scale environmental studies, from tracking deer populations to monitoring invasive aquatic species, making comprehensive data collection and analysis more feasible.
Douglas
at a Glance
Propulsion
6 (Surge, Sway, Heave, Roll, Pitch, Yaw)
T-200 Blue Robotics Thrusters (x8)
Hull and Chassis
Custom Aluminum Milled Hull
In-house machined and bent aluminum chassis
Custom Milled Blue Robotics Foam
Power System
Custom Power Governance PCB.
2 x 14.8V 20000mAH BlueRobotics LiPo Battery
Navigation Stack
SBG Elipse-N IMU
Water Linked A50 DVL
Custom Pressure Sensor
Computer Vision
Intel RealSense d455 (Forward Facing)
Arducam IMX219 Camera Module
Embedded Systems
Arduino Uno Rev3 (Propulsion).
Arduino Micro (Pressure Sensor)
Compute Module
NVIDIA Jetson AGX Orin
Machine Learning
PyTorch
YOLO v8
Software Stack
Robotics Operating System (ROS) Noetic
Ubuntu 20.04 LTS
Python3, C++, C
Mechanical Design.
Douglas features an entirely new mechanical body, designed and manufactured during the 2023-2024 scholastic year, consisting of a hull, chassis, and a grabber and dropper mechanism. Our mechanical team has rigorously tested numerous design iterations of components to ensure that Douglas can achieve the most optimal design.
Electrical Architecture.
Douglas has custom designed PCBs for regulating power, displaying system information on an LCD screen, transforming signals from the three hydrophones, and manipulating the grabber.
Software Stack.
Douglas has improved core software functionalities including a more precise navigation algorithm, development of advanced vision, and simulation algorithms.
Meet the AUV Team
Community & Outreach at McGill Robotics
McGill Robotics mission is to inspire students to build robots and to build robots that inspire students. We believe it is important to give back to the community which is why we are an organization that advances the field of robotics but also nurtures a community that values sustainability, collaboration, and education.